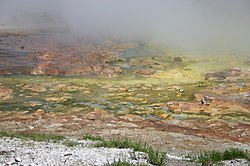
Archaea were once thought to be limited to extreme environments, but it has now been established that they are found in diverse ecosystems worldwide. Archaea may possess distinctive properties that affect biogeochemical processes, which makes understanding their distribution crucial in determining their effect on these processes. Little research has been done on the ecology of Archaea in. Install the Docker in your operating system. If you want to use PhySpeTree on other platforms, above all need to install docker on the appropriate platform. For Windows OS, you can install Docker for Windows. For Mac OS, you can install Docker for Mac. Pull the PhySpeTree image.
Previous:Phylogenetic TreeContentsSubsections
So far you have learned how to construct a phylogenetic tree using the structural alignment of AQPs.The sequence alignment can also be used to build a phylogenetic tree, especially when protein structures are notavailable.To make use of both the structural and sequence information,Multiseq now allows you to merge the two types of alignments and construct a completeevolutionary profile (EP) for the proteins being studied. In this section,you will learn how to obtain the EP for AQPs. For more information on using Multiseqprogram to perform evolutionary analysis, please refer to the Evolution of Biomolecular Structure tutorial.
Configure BLAST for Multiseq
For the following section you willneed to install BLAST on your computer. BLAST is a software that searchesthrough sequence databases and locate those sequences that are similar to aquery sequence. It is available online at http://www.ncbi.nlm.nih.gov/BLAST/ (click on Help tab, find and click theDownload link. At the bottom of the section titled 'Legacy executables,' clickon the link ftp://ftp.ncbi.nlm.nih.gov/blast/executables/release/LATEST/,and download BLAST as a guest). Here we will install a local copy of BLAST forMultiseq.- 2
- Extract the archives of BLAST.
- 1
- Open a new VMD and load the pdb files 1fqy, 1rc2, and 2f2b one by one.
- 3
- In the Multiseq program window, keep the protein structures under VMD Protein Structures and deleteall structures under VMD Nucleic Structures.
Load Sequences for AQPs in all three domains of life
Now that you have the structures of AQPs loaded, we will use BLAST to find sequences of AQPs in all three domains of life. Each of the three structures will be used as a query sequence by BLAST, and sequences in the swiss-prot database will be comparedwith them, one at a time. Those sequences similar to our query sequence will be picked by BLAST and loaded in Multiseq.- 3
- Choose for E Score and 1 for Iterations and then click OK.
- 5
- Click Accept. The sequences will be loaded in Multiseq.
Align Sequences Using a Structural Profile
In order to analyze the three structures and the 118 sequences of AQPs together, we need to first align them. What we will do is to first align the structures using the STAMP structural alignment tool mentioned in section 2, andthen we will use the structural alignment to guide the sequence alignments.- 2
- Click Tools Stamp Structural Alignment and choose to align Marked Structures and then click OK.
- 4
- Highlight all sequences and all structures as described above, so thatall sequences appear yellow and all boxes in front of sequence identifiers arechecked, then click Tools Sequence Alignment.
Construct an Evolutionary Profile for AQPs
Although we have obtained the structures and sequences for AQPs in all three domains and aligned them together, what we have now is notan evolutionary profile yet. We still need to get rid of the redundancy in these sequences caused by the biased databases.Multiseq provides a Sequence QR tool which can be used to select a minimum non-redundant set from the sequences, usinga threshold specified by the user.Archaea Terata Mac Os Download
Evolutionary profile provides an ``unbiased' view for the evolutionary relationship of the proteins in investigation. Using EP, scientists have successfullyidentified a new subfamily for the protein cysteinyl-tRNA synthetase. https://local-free-bet-iop-casino-tote-sport.peatix.com. For more details on constructing EP and performing evolutionary analysis, please referto the Evolution of Biomolecular Structure Top dollar slot. tutorial.
Next:Acknowledgements Up:Aquaporin Tutorial Previous:Phylogenetic TreeContentsschool@ks.uiuc.edu
Genesis of life on Earth told in core protein and DNA sequences: RNA polymerases reading DNA for content
To describe the genesis, divergence and complexity of life on Earth, one must understand two major transitions: 1) between the ancient RNA-protein world and LUCA (the last universal common ancestor); and 2) between LUCA and LECA (the last eukaryotic common ancestor). On Earth, life divides into three domains: bacteria, archaea and eukaryotes. Humans, plants and other organisms with complex cell and body structures are eukaryotes, so eukaryotes may have advantages in cell metabolism and developmental complexity compared to some single-celled organisms. Eukaryotes, however, result from chimeric fusion of multiple bacteria and at least one, now extinct archaea. Fused characteristics from bacteria and archaea license eukaryotes to support more complicated and rapid metabolism and increasingly intricate developmental programs.
Information flow within living organisms requires genetic material in DNA to be read and deciphered. Because, within the double helix, DNA bases project inward, DNA is more stable than single-stranded RNA, in which bases are more exposed. https://primebonusslotswbccodecasino-beautiful.peatix.com. LUCA, therefore, developed a more stable DNA genome than was possible in the RNA-protein world. The DNA double helix, however, is difficult to unzip, so proteins must act on DNA to separate strands. The relative security of a DNA genome compared to a fragmented RNA genome comes with conditions.
LUCA (~3.5 to 3.8 billion years ago) was one of the first organisms with a streamlined DNA genome. The Earth is only ~4.5 billion years old, so LUCA is very ancient and the time for the RNA-protein world that preceded LUCA was short. To read the genetic information within a DNA genome requires a mobile protein factory called RNA polymerase. To initiate RNA synthesis on a DNA template, RNA polymerases found in the three domains of life require protein factors that help them to recognize and open a promoter DNA sequence. In bacteria, sigma factors help RNA polymerases bind promoter DNA. In archaea, TFB (Transcription factor B) and TBP (TATA binding protein) cooperate to help RNA polymerase recognize a promoter. The evolutionary relationship, among bacterial sigma factors and archaeal TFB-TBP has not clearly been known.
Burton and Burton (2014)1 (son and father) demonstrate that bacterial sigma is related in evolution to archaeal TFB and eukaryotic TFIIB. Bacterial sigma factors have four helix-turn-helix (HTH) motifs, and archaeal TFBs have two related HTH motifs, which are common DNA-binding features of proteins. Burton and Burton hypothesize that both sigma factors and TFB arose from a primordial initiation factor at LUCA with 4-HTH motifs, and bacteria and archaea diverged in evolution about the time of LUCA largely because of this difference in genome reading styles. Archaeal TFB lost 2-HTH motifs and gained compensating functions including cooperation with TBP. Because bacteria and archaea interpret (read; 'transcribe') their DNA genomes differently to synthesize RNA, these organisms became distinct.
Evolution of promoter DNA sequences has long been a mystery, but bacterial promoters somewhat resemble archaeal and eukaryotic promoters. Evolutionary relatedness of bacterial sigma factors and archaeal TFB, however, generates a simple story about promoter evolution. According to this view, a primordial promoter was an AT-rich DNA sequence with a neighboring anchor DNA sequence. The anchor DNA sequence gives directionality to RNA synthesis by pointing RNA polymerase in the direction that it must read the gene. Because of chemistry of DNA bases, DNA strand separation is easier at AT-rich DNA than GC-rich DNA. Both bacterial sigma factors and archaeal TFB have a C-terminal HTH motif to bind the anchor DNA sequence, which remains double-stranded when the promoter DNA is opened closer to the start point for RNA polymerase to initiate a RNA chain and transcribe a gene. Another HTH motif helps to open up the DNA helix at or near a focused AT-rich DNA segment. So, evolutionary relatedness of bacterial sigma factors and archaeal TFB helps to describe the structure and evolution of directional DNA promoters with which these core protein factors interact. Anchor DNA sequences found in all of life are explained, and focused AT-rich DNA sequences common in promoters are explained from LUCA to the present.
Archaea Terata Mac Os X
Opinions and politics are influenced by what and ways humans read and access information (i.e. compare blinking in Fox News to reading the New York Times). Analogous to the stark divisions of modern American politics, very early in evolution, bacteria and archaea came to read and interpret genomic information in DNA in fundamentally different ways, causing bacteria and archaea to diverge into distinct domains. At LECA (~1.6-2.5 billion years ago) an archaea fused with multiple bacteria to form the first eukaryote. Sadly, it seems unlikely that a comparable synthesis of Republican and Democratic ideologies could be possible.
Understanding LUCA and LECA is key to describing divergence and evolution of life on Earth and also human complexity compared to single-celled organisms. The protein structures and sequences of sigma factors, TFB and multi-subunit RNA polymerases relate surprisingly straightforward stories about LUCA and LECA. Promoter evolution is also described. Essentially, protein structures and sequence comparisons reveal the most defining events in evolution of DNA-based organisms on Earth. In a preceding paper, a story of LECA and eukaryote complexity was recently told by Burton (2014).2
Because life evolved from a RNA-protein world and DNA originally came from RNA, the story of the genesis of life on earth is more easily told from the point of view of RNA polymerases rather than DNA polymerases.3 Strangely, bacterial and archaeal DNA polymerases are unrelated in evolution indicating that these essential mobile factories evolved separately after divergence. But exiting the RNA-protein world to LUCA must have initially involved RNA-dependent RNA polymerases and reverse transcriptases to synthesize DNA. If reverse transcriptase DNA synthesis mechanisms continued into LUCA, bacteria and archaea could have separately evolved distinct and unrelated DNA polymerases.
Glossary
- A, G, C, T:
- the bases that make up DNA
- Anchor DNA:
- the part of the promoter that remains double-stranded and points RNA polymerase in the appropriate direction to transcribe a gene
- DNA polymerase:
- a molecular machine to synthesize DNA
- HTH:
- helix-turn-helix motifs: a common DNA binding motif in proteins
- LUCA:
- last universal common ancestor
- LECA:
- last eukaryotic common ancestor
- Promoter:
- a DNA sequence from which RNA polymerase begins transcription of a gene
- Reverse transcriptase:
- a molecular machine to synthesize DNA from a RNA template
- RNA polymerase:
- a molecular machine that runs on DNA to polymerize RNA
- Sigma factor:
- a protein factor that directs initiation by bacterial RNA polymerase
- TFB:
- a protein factor that directs initiation by archaeal RNA polymerase
- TBP:
- TATA box binding protein
- Transcription:
- the process of RNA synthesis by RNA polymerase
References:
Archaea Terata Mac Os Catalina
Burton SP, Burton ZF. The sigma enigma: Bacterial sigma factors, archaeal TFB and eukaryotic TFIIB are homologs. Transcription 2014; 5:e967599.
Burton ZF. The Old and New Testaments of gene regulation: Evolution of multi-subunit RNA polymerases and co-evolution of eukaryote complexity with the RNAP II CTD. Transcription 2014; 5.
Koonin EV. The origins of cellular life. Antonie van Leeuwenhoek 2014; 106:27-41. Volvox mac os.